Open Positions: Motivated and molecular simulations inclined Ph.D. and M.Sc. students always welcome!
We shall not cease from exploration
And the end of all our exploring
Will be to arrive where we started
And know the place for the first time.
[ T. S. Eliot ]
News
April 3, 2024
In search for room temperature solvents for Birch reduction! In collaboration with colleagues and friends from the Fritz Haber Institute and the Helmholtz Center in Berlin and the University of Southern California, we used photoelectron spectroscopy and quantum chemistry calculations to characterized radical anions of naphtalene and benzophenone as key Birch intermediates in tetrahydrofurane. We showed that the stability of these intermediates depends primarily on the dielectric constant of the liquid environment paving way for designing optimal solvent mixtures for the Birch reduction process which would be more user-friendly than liquid ammonia.
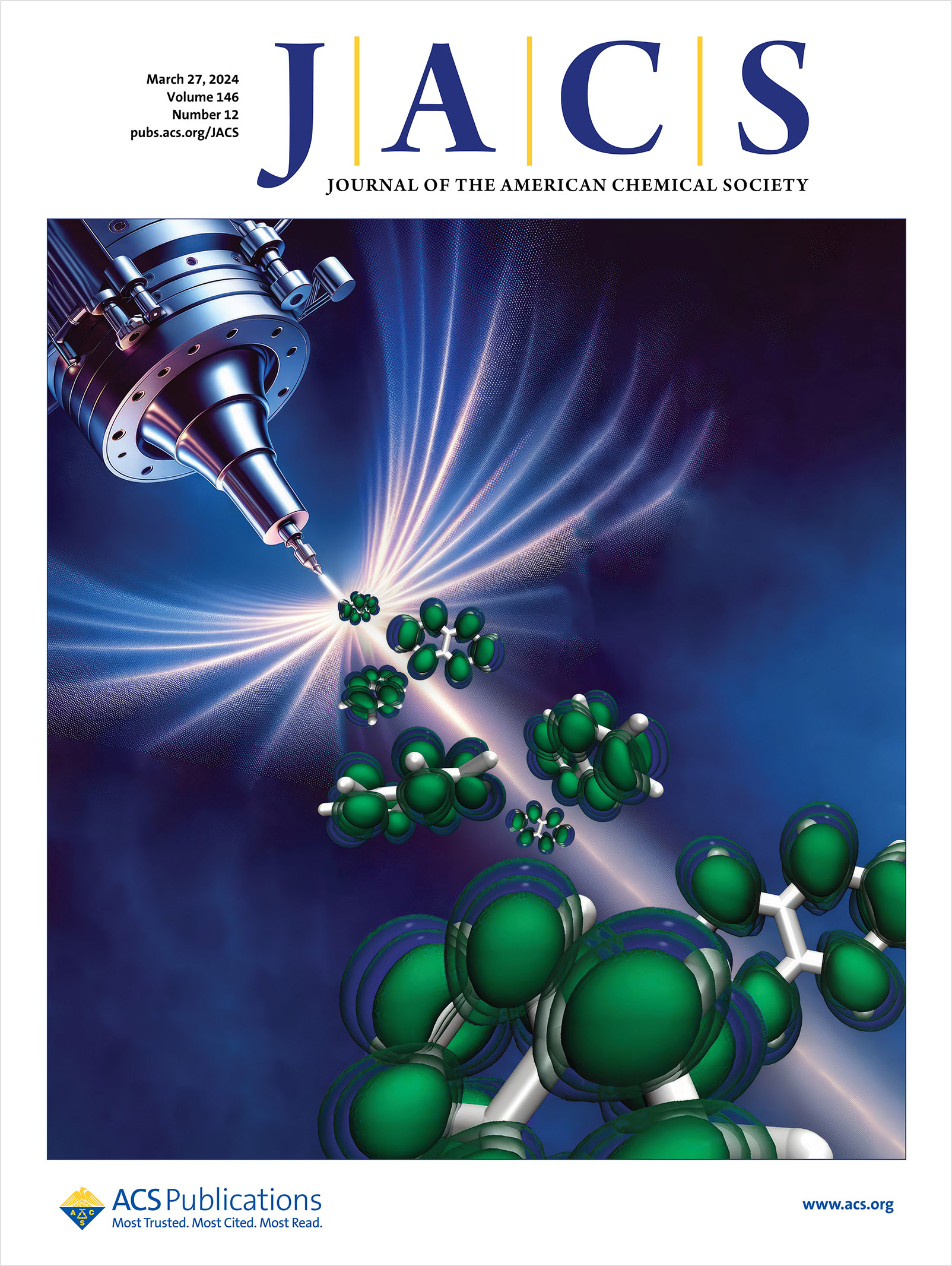
Issue cover: Artistic view of our experimental conditions: a liquid jet is generated from a cryogenic
nozzle setup into a vacuum while naphthalene molecules evaporate from the jet.
Nemirovich T., Young B., Březina K., Mason P.E., Seidel R., Stemer D., Winter B., Jungwirth P., Bradforth S.E., Schewe H.Ch.:
Stability and Reactivity of Aromatic Radical Anions in Solution with Relevance to Birch Reduction.
Journal of the American Chemical Society 146 (12): 8043–8057, 2024.
November 8, 2023
Coming out! After 12 years of work we are coming out with my extraordinarily talented and hard-working student Ondřej Ticháček and our friendly collaborator Pavel Mistrík at MED-EL Austria with a complete bottom-up computer model of the peripheral auditory system. In normal language this means that we can now model mammalian (yes, including human) hearing from the outer ear all the way to the auditory nerve. In other words, our model describes in a physically justified way and with physiologically relevant parameters how incoming sound translates to mechanical excitation in the middle and inner ear, followed by electrical excitation of the outer and inner hair cells and finally, via action of neurotransmitters to spikes in the auditory nerve, to be translated to the central nervous system. Why did I in 2011 start this project which seems totally out of the scope of research in our group? Mixture of craziness and personal reasons – a hard of hearing family member, as well as realization that at an atomistic level hearing is based on flows of ions like potassium and calcium, modelling of which is our daily bread. True, the present model does not go down to the atomistic level explicitly, but wherever possible builds in parameters reflecting the actual ionic flows in the inner ear. Our MATLAB-based model is now open for use to general public. So, whoever of you wants to play with it and, e.g., model various types of hearing impairment and in the future versions also model their compensation by hearing aids or cochlear implants, is welcome!
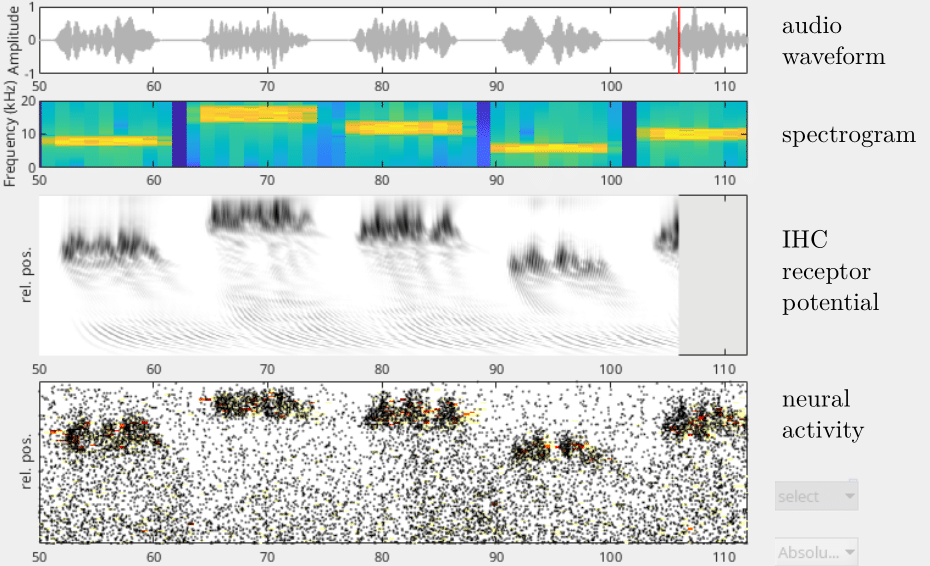
Graphical input/output of the model simulating a person saying the word "greasy" (don't ask me why this particular word).
Ticháček O., Mistrík P., Jungwirth P.:
From the outer ear to the nerve: A complete computer model of the peripheral auditory system.
Hearing Research 440: 108900, 2023.